- お役立ち記事
- Fundamentals and application points of quantum chemical calculations
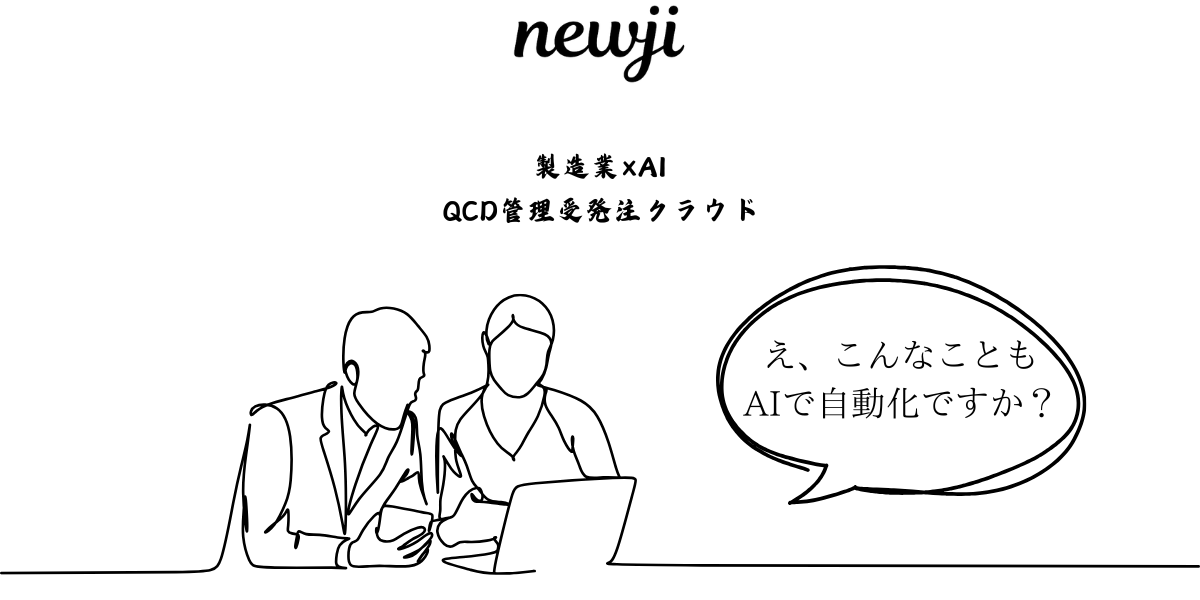
Fundamentals and application points of quantum chemical calculations
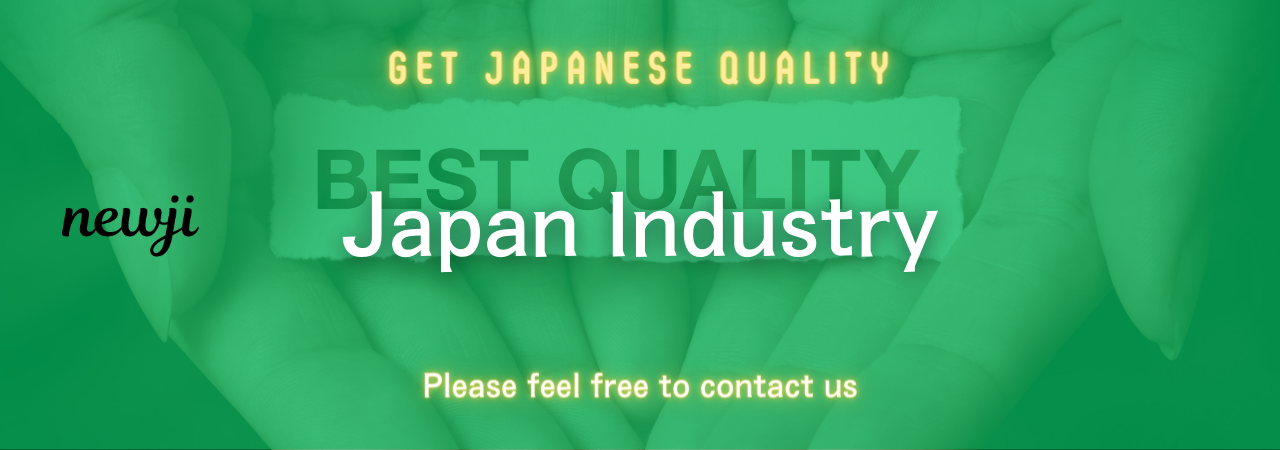
Understanding Quantum Chemical Calculations
Quantum chemical calculations are an essential tool in the world of chemistry, providing insights into molecular structures and reactions at the atomic level.
These calculations use quantum mechanics to model chemical systems, allowing scientists to predict the properties and behavior of molecules.
The fundamental principle behind quantum chemical calculations is that the behavior of electrons in atoms and molecules can be described using wave functions.
The calculations involve solving the Schrödinger equation, a mathematical formula that relates the wave function of a system to its energy.
This equation is central to quantum mechanics and helps in understanding how molecules form bonds and how they interact with each other.
Basic Concepts in Quantum Chemical Calculations
Before diving into the applications, it’s essential to understand the basic concepts involved in quantum chemical calculations.
First, the **wave function** is a mathematical expression that describes the probability of finding an electron in a particular region around the nucleus of an atom.
The square of the wave function gives the electron density, which can help visualize where electrons are likely to be located.
Next is the **Schrödinger equation**, which can be solved under various approximations to predict the energy and properties of molecules.
Since solving the equation exactly for all but the simplest systems is impossible, computational chemists use various methods to approximate solutions.
One of these methods is the **Hartree-Fock method**, which simplifies the Schrödinger equation by assuming that each electron moves independently in an average field generated by other electrons.
This method provides an initial approximation, but it often lacks accuracy for predicting chemical properties.
To improve upon Hartree-Fock, chemists use **post-Hartree-Fock methods**, such as Configuration Interaction (CI) and Coupled Cluster (CC) methods.
These account for electron correlation, making them more accurate but computationally demanding.
Applications of Quantum Chemical Calculations
Quantum chemical calculations have a wide range of applications across various fields.
In **materials science**, these calculations are used to design new materials with specific properties, such as increased strength or improved conductivity.
By understanding the electronic structure of materials, scientists can tailor their properties for specific applications.
In **drug discovery**, quantum chemical calculations help predict how new molecules will interact with biological targets, such as proteins or DNA.
This helps in designing more effective and safer pharmaceutical compounds, accelerating the drug discovery process.
The calculations are also essential in exploring **catalysis**, where chemists design catalysts to accelerate chemical reactions without being consumed in the process.
By understanding the reaction pathways and energy barriers, chemists can develop more efficient catalysts for industrial processes.
In **environmental science**, quantum mechanical methods are employed to study the atmospheric chemistry of pollutants and greenhouse gases.
Accurate predictions about chemical reactions in the atmosphere help develop strategies to mitigate pollution and understand the environmental impacts of chemicals.
Steps in Quantum Chemical Calculations
Performing a quantum chemical calculation involves several key steps.
First, researchers define the **geometry** of the molecule, specifying the positions of atoms, which serves as the starting point for the calculations.
Next, the appropriate level of theory is chosen based on the specific requirements of the study.
While the Hartree-Fock method offers a basic understanding, more complex problems might require Density Functional Theory (DFT) or post-Hartree-Fock methods.
Then, researchers select a **basis set**, which contains mathematical functions to describe atomic orbitals.
Larger basis sets generally provide more accurate results but require more computational resources.
The next step involves solving the Schrödinger equation using the chosen method and basis set to find the energy and wave functions of the molecule.
The calculations may involve **geometry optimization**, where the positions of atoms are adjusted to find the most stable structure with the lowest energy.
Finally, the results are analyzed to gain insights into the molecular properties or reaction mechanisms.
This might include interpreting the predicted energies, visualizing the electron density, or exploring potential energy surfaces to understand reaction pathways.
Challenges and Future Directions
Despite their utility, quantum chemical calculations face several challenges.
One of the primary challenges is the **computational cost**, as accurately modeling complex systems can require significant resources.
This is especially true for systems with many electrons or those involving heavy atoms, where relativistic effects must be considered.
Another challenge lies in the **trade-off between accuracy and computational efficiency**.
While more sophisticated methods provide better accuracy, they also demand more computational power and time.
Balancing these factors is crucial for practical applications.
In recent years, **advancements in computational hardware and algorithms** have significantly improved the efficiency and accuracy of quantum chemical calculations.
The development of machine learning models and new software tools promises to further enhance these calculations by enabling faster and more accurate predictions.
Conclusion
Quantum chemical calculations are a powerful tool for unraveling the complexities of atomic and molecular systems.
By applying quantum mechanics principles, these calculations provide invaluable insights into the behavior, properties, and interactions of molecules.
As technology advances, the capabilities of quantum chemical calculations continue to expand, offering even more precise and efficient solutions for a wide range of scientific challenges.
From materials design to drug discovery, their applications are vast, paving the way for innovations that impact various fields.
Ultimately, the ongoing development in this area promises exciting new opportunities for scientific discovery and technological advancement.
資料ダウンロード
QCD調達購買管理クラウド「newji」は、調達購買部門で必要なQCD管理全てを備えた、現場特化型兼クラウド型の今世紀最高の購買管理システムとなります。
ユーザー登録
調達購買業務の効率化だけでなく、システムを導入することで、コスト削減や製品・資材のステータス可視化のほか、属人化していた購買情報の共有化による内部不正防止や統制にも役立ちます。
NEWJI DX
製造業に特化したデジタルトランスフォーメーション(DX)の実現を目指す請負開発型のコンサルティングサービスです。AI、iPaaS、および先端の技術を駆使して、製造プロセスの効率化、業務効率化、チームワーク強化、コスト削減、品質向上を実現します。このサービスは、製造業の課題を深く理解し、それに対する最適なデジタルソリューションを提供することで、企業が持続的な成長とイノベーションを達成できるようサポートします。
オンライン講座
製造業、主に購買・調達部門にお勤めの方々に向けた情報を配信しております。
新任の方やベテランの方、管理職を対象とした幅広いコンテンツをご用意しております。
お問い合わせ
コストダウンが利益に直結する術だと理解していても、なかなか前に進めることができない状況。そんな時は、newjiのコストダウン自動化機能で大きく利益貢献しよう!
(Β版非公開)